Chemical Mechanisms of Enzyme Catalysis
How does an enzyme accomplish its tremendous enhancement of a reaction's rate (as much as a billion‐fold)? There is an upper limit to the activity of an enzyme: It cannot operate faster than the rate at which it encounters the substrate. In solution, this rate is approximately 10 8 to 10 9 times per second (sec ‐1). In the cell, enzymes acting on similar pathways are often located next to one another so that substrates don't have to diffuse away from one enzyme to the next—a mechanism that allows the enzymes to be more efficient than the theoretical limit. Even in solution, however, enzymes are powerful catalysts, and a variety of mechanisms bring about that power.
When a chemical reaction occurs, the energy content of the reacting molecule or atom increases. This is why most chemical reactions, whether they release heat or absorb heat, happen faster as the temperature is raised. The high‐energy state of the reactants is called the transition state. For example, in a bond‐breaking reaction, the transition state may be one where the reacting bond, although not completely broken, is vibrating at a frequency high enough that it is equally likely to split apart as to reform. Forming reactants or products results in the loss of energy from the transition state. This principle is shown in Figure , where the increased energy of the transition state is represented as a hill or barrier on the energy diagram. Catalysts reduce the height of the barrier for achieving the transition state.

Figure 1
What are the chemical mechanisms that enzymes use to make it easier to get to the transition state? Enzymologists have determined that a number of mechanisms seem to operate, including:
- Proximity. Enzymes can bring two molecules together in solution. For example, if a phosphate group is to be transferred from ATP to glucose, the probability of the two molecules coming close together is very low in free solution. After all, there are many other molecules that the ATP and the sugar could collide with. If the ATP and the sugar can bind separately and tightly to a third component—the enzyme's active site—the two components can react with each other more efficiently.
- Orientation. Even when two molecules collide with enough energy to cause a reaction, they don't necessarily form products. They have to be oriented so that the energy of the colliding molecules is transferred to the reactive bond. Enzymes bind substrates so that the reactive groups are steered to the direction that can lead to a reaction.
- Induced fit. Enzymes are flexible. In this regard, they are different from solid catalysts, like the metal catalysts used in chemical hydrogenation. After an enzyme binds its substrate(s), it changes conformation and forces the substrates into a strained or distorted structure that resembles the transition state. For example, the enzyme hexokinase closes like a clamshell when it binds glucose. In this conformation, the substrates are forced into a reactive state.
- Reactive amino acid groups. The side chains of amino acids contain a variety of reactive residues. For example, histidine can accept and/or donate a proton to or from a substrate. In hydrolysis reactions, an acyl group can be bound to a serine side chain before it reacts with water. Having enzymes with these catalytic functions close to a substrate increases the rate of the reactions that use them. For example, a proton bound to histidine can be donated directly to a basic group on a substrate.
- Coenzymes and metal ions. Besides their amino acid side chains, enzymes can provide other reactive groups. Coenyzmes are biomolecules that provide chemical groups that help catalysis. Like enzymes themselves, coenzymes are not changed during catalysis. This distinguishes them from other substrates, such as ATP, which are changed by enzyme action. Coenzymes, however, are not made of protein, as are most enzymes. Metal ions can also be found in the active sites of a number of enzymes, bound to the enzyme and sometimes to the substrate.
Coenzymes provide chemical functional groups that proteins lack. For example, only sulfhydryl groups on amino acids are able to participate in oxidation and reduction reactions, and the formation/breakage of disulfides does not provide enough reducing power to alter most biomolecules's functional groups. Electron transfer requires one of several coenzymes, usually either nicotinamide adenine dinucleotide, NAD, or flavin adenine dinucleotide, FAD, as electron acceptors and donors. Table shows some of these coenzymes.
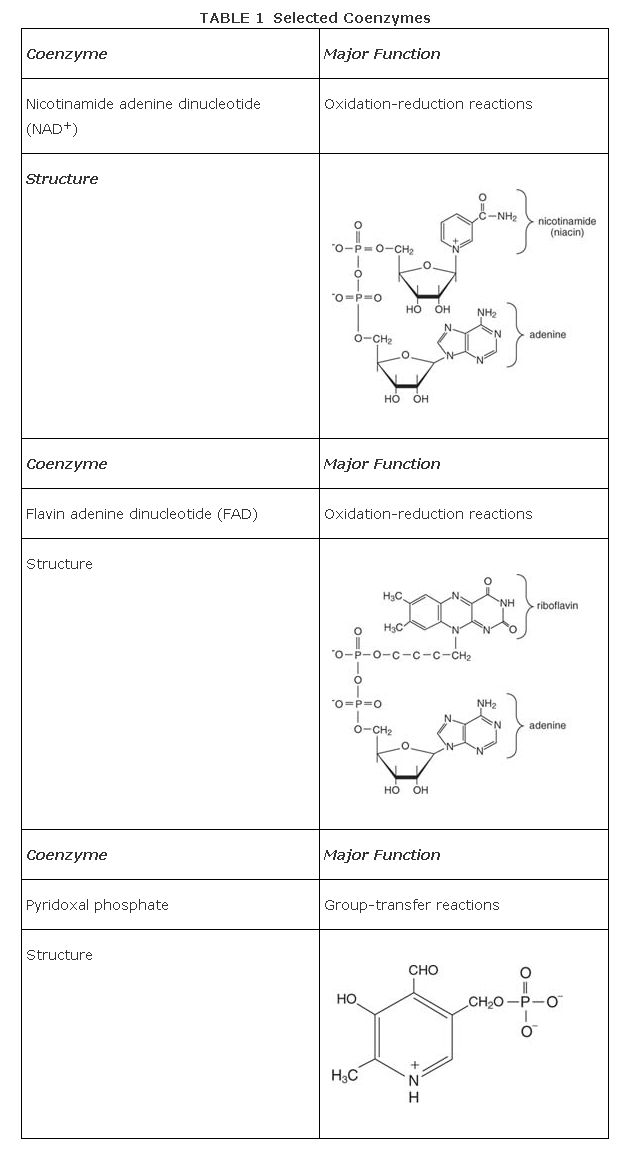
Some coenzymes participate in group‐transfer reactions that are difficult to carry out with amino acid side chain chemistries alone. For example, none of the side chains of the normal 20 amino acids can accept an amino group easily. On the other hand, the coenzyme pyridoxal phosphate has a carbonyl group that is well adapted to accepting or donating amino groups.
Vitamin conversion
Vitamins are organic compounds required for human and animal growth. Many microorganisms (although by no means all) can grow and reproduce in a simple medium of sugars and inorganic salts. Likewise, photosynthetic organisms can synthesize all the organic molecules needed for life. These organisms don't need vitamins because they can synthesize them from simpler chemicals.
Our species has lost the ability to make vitamins. Thus, deficiency of niacin (nicotinamide), the “N” in NAD, leads to the disease pellagra, a collection of skin, intestinal, and neurological symptoms. (Niacin can be synthesized from the amino acid tryptophan, so pellagra results from a deficiency of both niacin and tryptophan in the diet.)